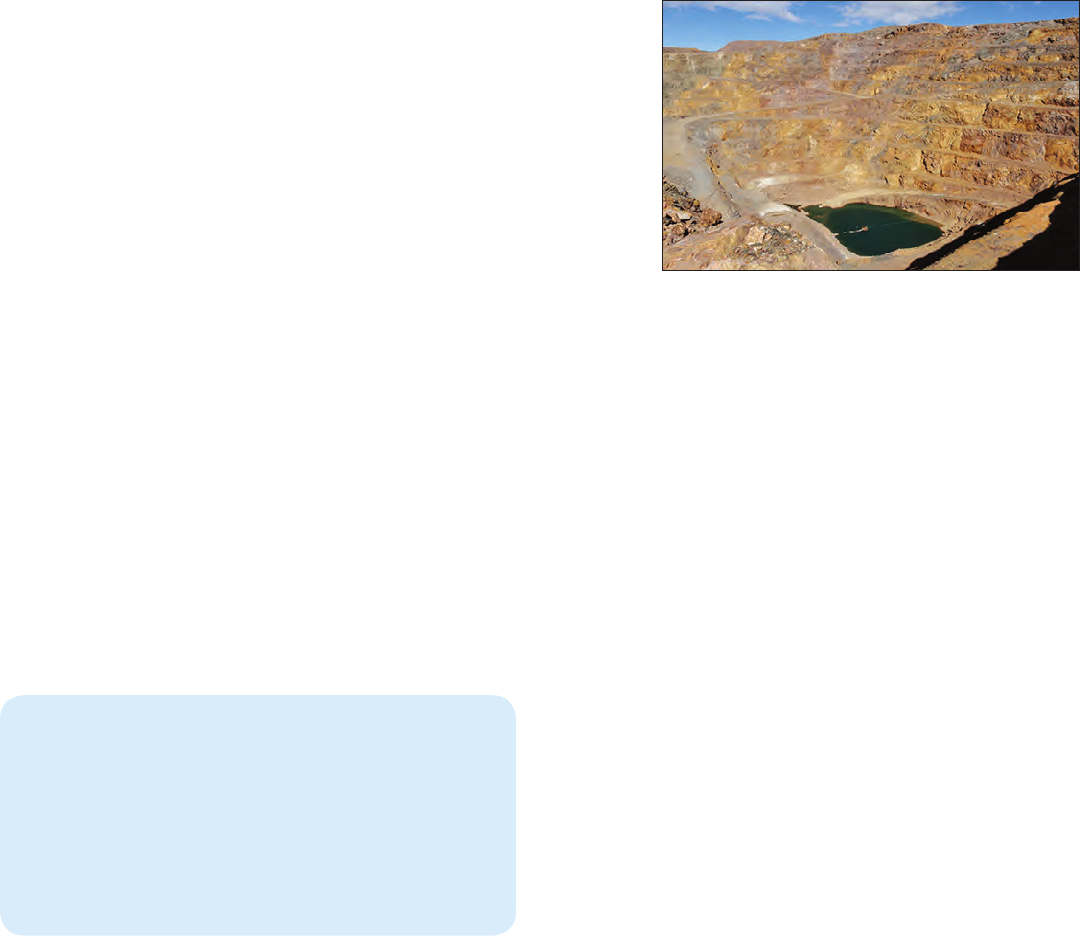
Worldwide Supply and Demand for Rare-Earth Elements
As noted earlier, in recent years Chinese production has accounted for
about 95 percent of the REE global market. Citing a need to retain their limited
REE resources for domestic requirements and concerns for environmental effects
of mining, China has restricted the supply of REEs through quotas, licenses, and
taxes. As a result, the REE industry outside of China increased REE stockpiling,
explored for deposits in many locations, and promoted new efforts to conserve,
recycle, and nd substitutes for REEs. New mine production has begun in
Australia (Mount Weld) and the United States (Mountain Pass, California).
In recent years, expert panels convened by research institutes and
government agencies highlighted specic REEs as raw materials critical to
evolving technologies, such as clean-energy applications, high-tech military
components, and electronics (Long and others, 2010). These reports suggest
that a high potential exists for disruptions in REE supplies. As a result,
several expert panel analyses rank REEs high on the “criticality” factor of
raw materials, meaning they are of high technological and economic impor-
tance and have high supply-side risk (National Research Council, 2008). Panels and agencies that assessed the criticality of REEs and
other raw materials include the National Research Council, U.S. Department of Energy, European Commission, American Physical
Society (APS) and Materials Research Society (MRS), and the Resnick Institute.
Worldwide explorations for economic deposits of REEs and efforts to bring them into production have increased substantially
since 2000. More than 400 rare-earth projects were in progress during 2012, including many projects in advanced stages of explora-
tion, meaning that the deposit sizes and REE concentrations were announced based on detailed drilling. One important aspect in the
development of a property for REE mining is the cost and complexity of processing the REE ores. Recovery of REEs can be complex
because they occur in minerals as a group of similar elements, and at many deposits the REEs are hosted within more than one
mineral. Not only do REE-rich minerals need to be concentrated, but the actual elements must be separated from each other, usually
as oxide compounds (for example, lanthanum oxide). The success and timeliness of rare-earth mining projects, and the rare-earth
elements industry in general, is difcult to predict and will be continuously monitored and studied by the USGS.
References
Lide, D.R., ed., 2004, Abundance of elements in the Earth’s crust and
in the sea, in sec. 14 of CRC handbook of physics and chemistry—
A ready-reference book of chemical and physical data (85th edition):
Boca Raton, Fla., CRC Press, p. 17 [table].
Long, K.R., Van Gosen, B.S., Foley, N.K., and Cordier, Daniel,
2010, The principal rare earth elements deposits of the United
States—A summary of domestic deposits and a global perspective:
U.S. Geological Survey Scientic Investigations Report 2010 –5220,
96 p. [Also available at http://pubs.usgs.gov/sir/2010/5220/.]
National Research Council, 2008, Minerals, critical minerals, and the
U.S. economy: Washington, D.C., National Academies Press, 264 p.
[Also available at http://www.nap.edu/openbook.php?record_id=12034.]
For More Information
On production and consumption of rare-earth elements:
http://minerals.usgs.gov/minerals/pubs/commodity/rare_earths/
On historical statistics of rare-earth elements:
http://minerals.usgs.gov/ds/2005/140/
On end use and recycling of rare-earth elements:
http://pubs.usgs.gov/sir/2011/5094/
The USGS Mineral Resources Program is the sole Federal provider
of research and information on rare-earth elements and other nonfuel
mineral resources. For more information, please contact:
Mineral Resources
Program Coordinator Telephone: 703-648-6100
U.S. Geological Survey Fax: 703-648-6057
Reston, VA 20192 Home page: http://minerals.usgs.gov
Text prepared by Bradley S. Van Gosen, Philip L. Verplanck,
Keith R. Long, Joseph Gambogi, and Robert R. Seal II.
The Mountain Pass mine of Molycorp, Inc., southeastern
California, is the only active producer of REEs in the United
States (2014). The ore body is a carbonatite intrusion,
thought to be the largest REE resource in the United States.
How Do We Ensure Adequate Supplies of
Rare-Earth Elements for the Future?
Global REE resources are estimated to be 110 million
metric tons of rare-earth oxide, which primarily occur, in
descending order, in China, Russia, the United States, India, and
Australia. Exploration for additional REE deposits is ongoing;
therefore, the world’s known in-the-ground resources (endow-
ment) of REEs are likely to increase. Despite many known REE
deposits, the global supply of REEs is limited by the cost and
complexity of exploring REE deposits and developing REE
mines, including REE extraction and separation facilities. These
factors are discussed in USGS Scientic Investigations Report
2010 –5220 (available at http://pubs.usgs.gov/sir/2010/5220/).
In addition to bringing more REE deposits into produc-
tion, other methods may help offset REE supply restrictions.
Examples include new efforts to recycle REEs, research to nd
substitute materials for REEs, and efforts to recover REEs as
coproducts of mineral deposits. These efforts may eventually
offset some of the demand for REEs.
ISSN 2327– 6932 (online)
http://dx.doi.org/10.3133/fs20143078
Did you know....
In the 1940s, as part of the Manhattan Project that
created the nuclear bomb, Frank Spedding and others in the
United States developed chemical ion exchange procedures
that could separate and purify individual REEs. This method
was first used to separate plutonium-239 and neptunium
from uranium, thorium, and actinium in materials generated
by nuclear reactors.